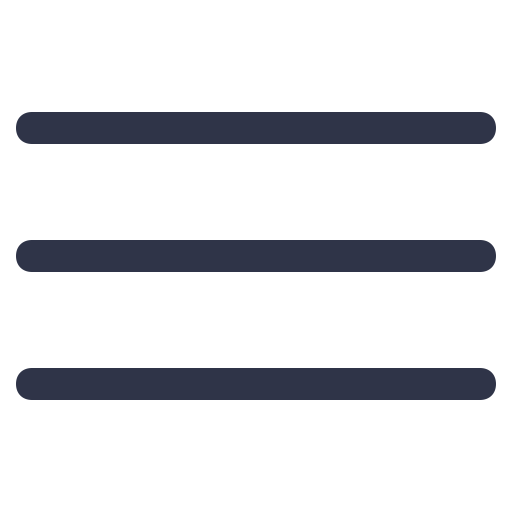
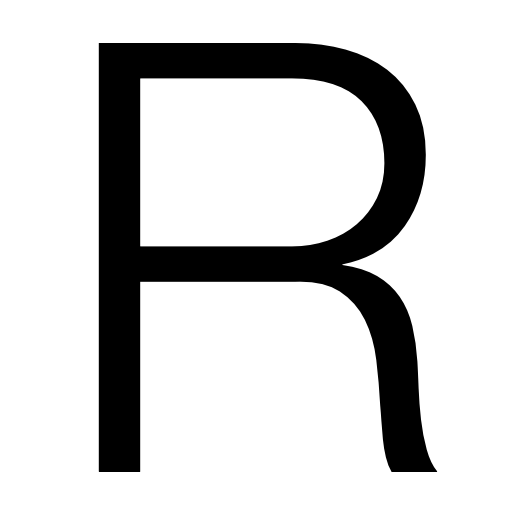
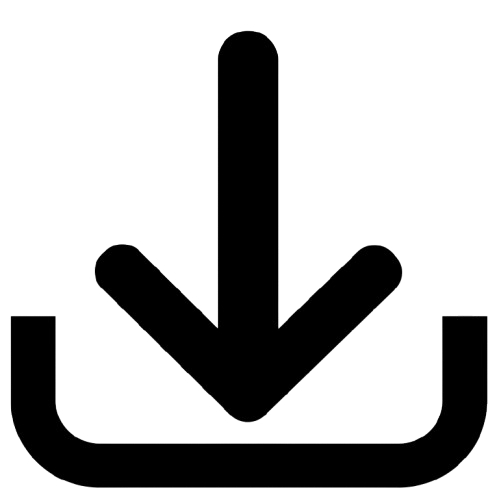
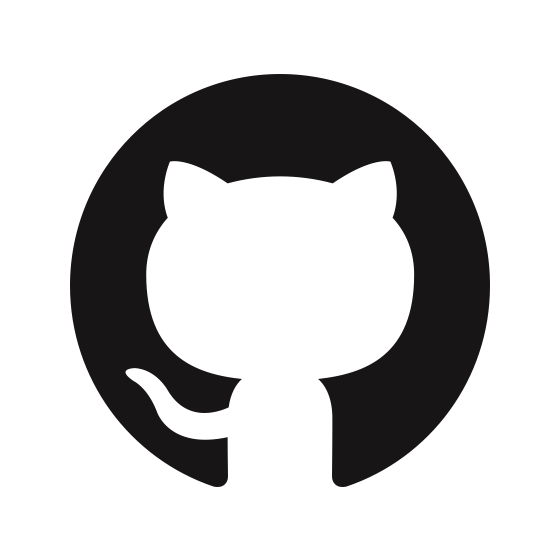
6.5 The Phase-2 Upgrade
6.5.1 Tracker
In the HL-LHC, the tracker will have to endure much higher radiation levels and help mitigate the larger 140–200 expected pileup interactions. The entire CMS tracker will thus be replaced during the long shutdown 3 (LS3) between 2026 and 2029 with the “Phase-2” tracker [43], comprised of an Inner Tracker of silicon pixels and an Outer Tracker of silicon strip and “macro-pixel” modules. Overall, the upgrade will improve its radiation hardness, granularity, as well as increase its forward acceptance.
A key additional novelty is the inclusion of dedicated “ modules” [199] in the Outer Tracker to efficiently and quickly detect high () tracks. This will allow, for the first time, L1 trigger decisions to be based on tracker information. This is crucial to mitigate the increased pileup, and may perhaps even improve the trigger efficiency for objects such as -jets and -leptons [200].
6.5.2 Timing layers
The Phase-2 upgrade of CMS will include a novel, thin layer of timing detectors between the tracker and calorimeters to provide a target resolution of 30–60 for charged particles. This precise timing information will be crucial for reducing OOT pileup particles not compatible with the time of the primary vertex, with an estimated effective pileup reduction from 200 to between 33–70 [201]. It may additionally aid particle identification (PID), and hence jet tagging, using time-of-flight measurements to calculate particle velocities and masses for given momenta [202].
The timing layers are based on minimum ionizing particle (MIP) timing detectors (MTDs). MIPs are high-energy particles which deposit a small fraction of their energy as they traverse and ionize the sensors. MTD sensors are designed for rapid signal collection and response to these interactions to achieve the target timing resolution. Two separate barrel and endcap timing layers (BTL and ETL, respectively) will be installed, using different sensor technologies based on the different geometries and radiation levels.
The BTL will be made out of 300,000 scintillating Cerium-doped Lutetium (LYSO) crystals, known for their fast response time and high light yield, read out by silicon photomultipliers (SiPMs), also known for speed and good photon detection efficiency (PDE). This combination has been measured to provide the desired time resolution of in charged pion test beams [203]. Both the crystals and SiPMs are sufficiently radiation-hard for the barrel; however, SiPM PDE is expected to degrade over time due to increased dark current noise, reducing the timing resolution to about - by the end of the HL-LHC [203].
The more extreme levels of radiation in the endcap preclude the use of SiPMs. Instead, the ETL will use a more radiation-hard silicon sensor known as a low gain avalanche detector (LGAD). LGADs incorporate an extra gain layer into the typical - junction diode in order to rapidly amplify the signal by a factor of –. They have been measured to allow single-hit resolution for MIPs at the level of –, even after the full expected radiation dose of the HL-LHC [201].
6.5.3 Barrel calorimeters
In the barrel, the PbWO crystals and photodiodes of the ECAL are expected to perform well and will be retained, although the operating temperature will be lowered from to C to counter increased noise in the photodiodes [44]. The electronics will be upgraded to be faster and more radiation tolerant, with a target time resolution of for energy deposits greater than to mitigate pileup. The radiation damage to the HCAL barrel active material is expected to have a negligible impact on the physics performance and, hence, the HB scintillators and fibers will also be retained [204]. However, its back-end electronics will be similarly upgraded to sustain the higher L1-trigger rate.
6.5.4 HGCAL
Both the ECAL and HCAL calorimeter endcaps (CEs) will be replaced entirely by the new High-Granularity Calorimeter (HGCAL) [205] due to the extreme forward radiation levels expected. The HGCAL has been designed to not only withstand the increased radiation, but also to provide: (1) high lateral granularity, for better shower separation and narrow jet identification; (2) fine longitudinal granularity, for better shower shape and energy resolution; as well as (3) precision timing for pileup rejection. The latter means HGCAL will be a 5D calorimeter, able to measure the position, energy, and timing of hits.
The HGCAL will be a large sampling calorimeter with a total of 47 absorber and sensor layers, illustrated in Figure 6.15. The electromagnetic section (CE-E) will comprise 26 sensitive layers of 0.5–1 silicon sensors interleaved with copper, copper-tungsten, and lead absorber plates, with a total thickness of and . The hadronic section (CE-H) will contain 21 layers of sensors, with silicon in the high-radiation regions and 4–30 plastic scintillating tiles read-out by SiPMs in the low-radiation regions (Figure 6.16), and stainless steel and lead absorbers, for a total thickness of . The entire HGCAL will be operated at C to keep electronic noise sufficiently low.
Silicon is again chosen for the benefits described in Section 6.3.2, as well as its fast response time, which is expected to provide time resolution of depending on the energy of the hit. This has been shown to significantly aid in pileup rejection [206]. Cheaper plastic scintillators are used where the radiation levels are lower and, additionally, a hexagonal geometry is chosen to cover the more than 600 of silicon area required in the most cost-effective manner, as shown in Figure 6.16.
Overall, the high-granularity, high-density, and fast timing calorimetry of the HGCAL is expected to significantly improve electron, photon, and jet efficiency and resolution, mitigate pileup, and allow more powerful trigger algorithms in HL-LHC [205]. However, the increased complexity and occupancy, and unorthodox geometry, will pose significant challenges not only in its design and construction, but also computationally with respect to its simulation and reconstruction. This is a strong motivation for the exploration of new computing techniques for fast and efficient CMS simulations, as we will discuss in Part IV.
6.5.5 Muon system
As with the barrel calorimeters, the gas detectors themselves in the muon system are expected to continue performing well at the HL-LHC with no significant degradation in the overall muon reconstruction performance [47]. The electronics, however, will be upgraded to be more radiation hard and sustain the higher trigger rate.
The higher radiation and pileup does pose considerable challenges to reliable muon triggering in the very forward regions, however. In fact, trigger inefficiencies were already anticipated in Run 3 of the LHC, which led to the installation of a new gas electron multiplier (GEM) station in the endcap regions of the muon system in the 2019–2022 long shutdown before Run 3, and recently another GEM station at the beginning of 2024 [207]. GEMs are popular gas detectors with high rate capability and radiation tolerance, and provide crucial additional hit information to improve the trigger efficiency and muon reconstruction in the forward regions.
During LS3, two more RPC stations for and one final GEM will be added in the endcap regions for HL-LHC. The RPCs will provide further forward timing information as well to complement the CSCs and recover single-muon trigger efficiencies [208].